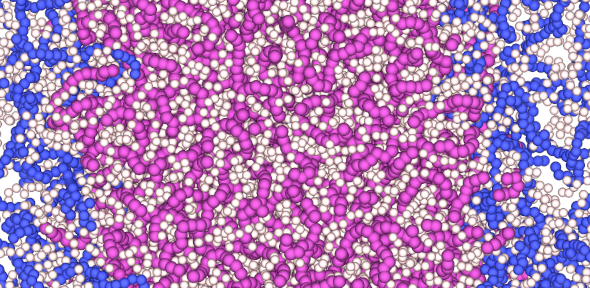
A mixture of proteins (in magenta and blue) forming a multi-layered compartment. Credit Pin Yu Chew & Aleks Reinhardt
Researchers here have created a model which can describe and predict how biomolecules self-organise into compartments inside living cells, leading the way towards a better understanding of the causes of some neurodegenerative diseases and cancers.
In the paper published in Nature Computational Science, researchers from the University of Cambridge Departments of Chemistry, Physics and Genetics describe how their new model, dubbed Mpipi [for Multiscale π–π], achieves a new level of accuracy and testability in describing and predicting biomolecular phase behaviour.
Just like our planet, whose equilibrium depends on multiple interactions between climate, geology, animals and plants, a cell can be viewed as a tiny world inhabited by a variety of species, whose interactions affect its performance.
These cell inhabitants, known as biomolecules, are made up of proteins and nucleic acids. And just as on Earth, they form diverse microenvironments with distinct conditions, coordinating thousands of simultaneous chemical reactions.
“Cells form compartments with distinct local microenvironments in which different molecules are present,” said Dr Aleks Reinhardt, joint first author of the study, whose group in this Department develops computer simulations to study the behaviour of molecular and colloidal systems. “Concentrating specific molecules and excluding others in different ways in various parts of the cell allows the compartments to fulfil a wide range of biological functions.”
Some biomolecules form membrane-bound organelles, which are essentially the cell nucleus and mitochondria bound by a membrane. However, the most widespread form of compartments within cells completely lack membranes, and are known as biomolecular condensates. These compartmentalised biomolecules are sustained and segregated in space by the physical chemistry of liquid–liquid phase separation (LLPS), which is analogous to oil droplets dispersed in water.
LLPS in cells is thought to have wide-ranging implications, such as gene silencing and gene activation. But sometimes these biomolecular condensates malfunction, and that has been linked to neurodegenerative disorders such as amyotrophic lateral sclerosis (the disease notably associated with Stephen Hawking) and cancer.
This link has motivated major efforts to understand the principles that underlie biomolecular interactions within cells. Over the past three years, many computational models have been developed to interpret and predict these interactions.
“Because LLPS is a collective phenomenon, which emerges from the interactions among thousands of proteins and nucleic acids, molecular modelling and simulations have surfaced as powerful tools that can link the physicochemical features of individual biomolecules to their phase behaviour,” said senior author Dr Rosana Collepardo-Guevara, whose group develops multiscale modelling approaches to investigate the driving forces of biomolecular self-organisation within cells.
The new Mpipi model provides a quantitative description of how protein phase diagrams are modulated against specific amino-acid sequence changes in agreement with experiments and, therefore, can be used to understand what amino-acid interactions dominate the phase behaviour.
“A key feature of our model is that it carefully balances the contribution of each of the different amino acids to protein LLPS by combining information from detailed all-atom simulations and statistical data from large databases of known protein structures,” said Dr Jerelle Joseph, joint first author and Junior Research Fellow at King’s College.
The team benchmarked the performance of Mpipi against seven other leading sequence-dependent coarse-grained models, which together represent the state-of-the-art in the field. They also tested the predictions of their approach by comparing them with experimental single-molecule radii of gyration and directly with experimental phase diagrams of proteins as a function of temperature and protein concentration. The tests demonstrated the Mpipi model’s excellent agreement with experiment.
“I believe that in order to understand how cells work in both health and disease, advances in computer models are as important as those in experimental techniques. Partnering accurate molecular models like Mpipi with modern experimental techniques holds great potential for changing our views of the fundamental mechanisms that regulate membrane-less compartments within cells,” said Collepardo.
“The ability to decipher how these biomolecular condensates behave is critical to predict and rationalise their stability not only when they occur in healthy organisms, but also to eventually design strategies to dissolve them when aberrant conditions might lead to disease.”
Research
Physics-driven coarse-grained model for biomolecular phase separation with near-quantitative accuracy, J. A. Joseph, A. Reinhardt, A. Aguirre, P. Y. Chew, K. O. Russell, J. R. Espinosa, A. Garaizar and R. Collepardo-Guevara, Nat. Comput. Sci., (2021).
Access this paper as part of the Springer Nature Content Sharing Initiative.