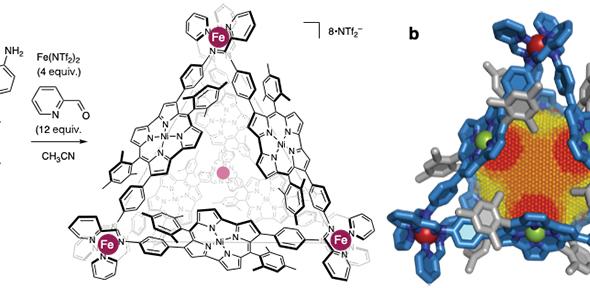
Researchers working in the Nitschke group here have successfully created the first self-assembled nanocage to have antiaromatic walls.
In doing so, they have overturned assumptions about the limits of nano-chemical engineering and created an entirely new nanospace for scientists to explore.
Nanocages – nanometre-sized cavities – are complex, functional structures that are already being used in a range of applications in chemistry, medicine and environmental science.
In the Nitschke group, for example, researchers work to design hollow supramolecular capsules or 'cages' that can be employed to collect, transport and deliver cargoes of molecules to where they are needed. These could have a use in safely delivering drugs within the body, or replacing the current high-cost, high-energy processes used in chemical purification and petroleum refining.
But while many research teams have so far developed nanocages with aromatic walls, none have yet done so with antiaromatic compounds because of the challenges posed by their inherent instability.
Today, however, a team of scientists – including Masahiro Yamashina of the Tokyo Institute of Technology, Michael Pittelkow from the University of Copenhagen and Jonathan Nitschke here in the University of Cambridge Department of Chemistry – report in the journal Nature their work in creating "a self-assembled cage composed of four metal ions with six identical antiaromatic walls".
The work was completed while Dr Yamashina was working in the Nitschke group as an Overseas Research Fellow of the Japan Society for the Promotion of Science.
The breakthrough opens up new lines of research in this field. Aromaticity and antiaromaticity, as the paper explains, are fundamental concepts in chemistry and the preparation of antiaromatic molecules and the study of their properties has been of longstanding interest to chemists, but also of longstanding difficulty.
Aromaticity refers to a property of ring-shaped organic compounds that makes them highly stable, whereas antiaromaticity describes compounds that are far more reactive, due to a difference in the number of so-called pi-electrons shared by the ring.
"The idea of constructing an antiaromatic cage is one we have been looking at for several years," says Professor Nitschke, corresponding author on the paper. "But the synthetic process involved is extremely arduous and challenging and a previous attempt that was made here using a different method failed.
"Masahiro had to overcome a great many blind alleys and difficulties during this work. But he was very persistent and now that he’s succeeded, he has opened a door to scientists who want to explore the area further."
The team's search for a suitable building block for their antiaromatic nanocage led them to a 2012 study by Hiroshi Shinokubo and co-workers in Japan. This study reported the synthesis of an unusually stable, nickel-based antiaromatic compound called norcorrole.
Then, drawing on the expertise of Professor Nitschke and his group in subcomponent self-assembly, the researchers succeeded in building a three nanometre-diameter cage with a norcorrole skeleton.
They then tested the degree of antiaromaticity within the cage and the unique environment inside it. They encapsulated a number of guest molecules within this new cage and discovered, as they had predicted, that when exposed to an external magnetic field – for example, inside a nuclear magnetic resonance (NMR) spectrometer – these molecules experienced a de-shielding effect.
The antiaromatic-walled cage can thus be considered a new type of NMR shift reagent, the researchers say, meaning that it could be a tool for analysing and interpreting the fine structures of organic compounds.
NMR spectroscopy is a technique used to observe local magnetic fields around atomic nuclei. The sample is placed in a magnetic field and the NMR signal is produced by excitation of the nuclei sample with radio waves into nuclear magnetic resonance. The intramolecular magnetic field around an atom in a molecule changes the resonance frequency, thus giving access to details of the electronic structure of a molecule.
"If we put a guest molecule into one of these antiaromatic cages into an NMR spectrometer," says Professor Nitschke, "it de-shields them – i.e. it moves the signals of the molecule away from where they ordinarily are, and away from where they might overlap with other signals and you can clearly see what is going on inside them.
"We have done this for fairly simple molecules. But we’d like to be able to do it for more complex molecular systems where there are a lot of signals and they tend to lie on top of one another. We have previously encapsulated proteins – which are large, complex molecules – inside aromatic cages. If we could now encapsulate a protein inside an antiaromatic cage, it would give us a much clearer picture of what is going on inside it."
"We have not been able to do this in the past. But we could now and this advance opens up a whole new space to explore."
- References: Masahiro Yamashina, Yuya Tanaka, Roy Lavendomme, Tanya K Ronson, Michael Pittelkow & Jonathan R Nitschke. ‘An antiaromatic-walled nanospace’. Nature (2019). DOI: 10.1038/s41586-019-1661-x
- This study was supported by the European Research Council and the UK Engineering and Physical Sciences Research Council.
- Main image: the chemical structure of an antiaromatic-walled nanospace. (a) construction of antiaromatic-walled nanospace. (b) X-ray crystal structure with a 3D NICS grid, showing magnetic deshielding experienced within the nanospace. Antiaromaticity becomes stronger in the order of yellow < orange < red.
- Smaller image: the project team in the Nitschke group. From left to right: Dr Tanya K Ronson, Dr Masahiro Yamashina, Professor Jonathan R. Nitschke and Dr Roy Lavendomme.