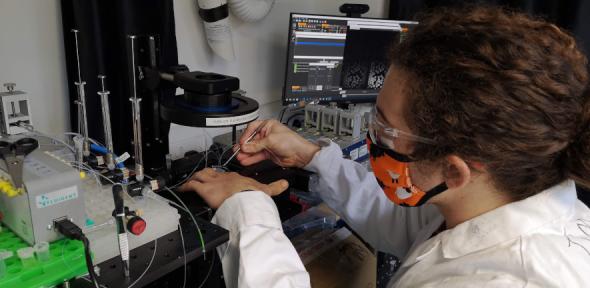
New research from the Knowles and Collepardo labs has implications for therapies against cancer and other diseases, and could even contribute to theories about the origin of life.
In a paper published in Nature Communications, a multi-disciplinary team with lead co-authors Dr Georg Krainer, Timothy Welsh and Dr Jerelle Joseph, has shown for the first time that liquid-like protein compartments, known as condensates, can re-enter a phase-separated regime in response to different salt concentrations. This discovery may help develop future therapies for cancer and neurodegenerative diseases, and may even have implications for the origin of life on earth.
Understanding aberrant condensates in cells
Cell interiors contain a myriad of proteins and other biomolecules which must be carefully organised in space for the cell to function correctly. To do this, the nucleus and other well-known cellular compartments enclose groups of proteins with membranes, but membrane-less compartments known as biomolecular condensates are also common.
Condensates are protein-rich liquid drops that remain segregated through the physics of liquid–liquid phase separation, which is analogous to the separation of oil and water into distinct liquid phases. The advantage of these phase separated compartments or condensates over their membrane-bound counterparts, is that the cell can dynamically trigger their formation and dissolution simply by changing its environment.
However, sometimes these normally useful cell components transition into malfunctioning or ‘aberrant’ condensates, which tend to display different chemical and physical properties than healthy ones. These ‘bad’ condensates have been implicated in different forms of cancer and neurodegenerative diseases such as Alzheimer’s disease and Amyotrophic lateral sclerosis (ALS).
In order to understand the process of protein phase separation further, researchers from the Knowles and Collepardo labs here, working together with groups from the Technical University in Dresden, the Max Planck Institute of Molecular Cell Biology and Genetics, and the University of Toronto, investigated how the liquid phase separation of certain proteins is affected by different concentrations of ionic salts.
The researchers discovered that some proteins form condensates under low-salt conditions, then turn into a well-mixed state under medium-salt concentrations, but transition once again into a condensate-forming regime at higher concentrations of salt.
From left: Dr Georg Krainer, Dr Jerelle Joseph, and postgraduate student Timothy Welsh.
Reentrant phase transition
This behaviour is known as ‘reentrant phase transition’ because the proteins have gone from a phase-separated state to a diluted, well-mixed solution and then re-entered the phase-separation state. “Reentrant phase separation for proteins has been reported before in multicomponent systems; for example, by changing the concentration of RNA. Our work shows that proteins can also re-enter the phase-separated regime simply by variations in salt concentration,” says postdoctoral researcher Dr Jerelle Joseph. “We knew that the low salt phase separated regime existed, but it was not clear that the reentrant phase transition at high salt concentration occurred.”
Postdoctoral researcher Dr Georg Krainer, explains further: “Other researchers have shown a transition from no condensate to a condensate and back. But now we showed that this reentrant phase separation can occur without the aid of additional molecules such as RNA. This means we have shown that the transition happens because of the intrinsic properties and interactions of the protein itself in response to its changing environment.”
The team demonstrated that the molecular interactions which drove the phase transitions in the low and high salt regimes were fundamentally different. Krainer says: “In the low-salt regime, hydrophobic and electrostatic interactions are important, but in the high salt regime the condensates needed hydrophobic and non-ionic interactions to form.”
This discovery has implications in creating drug therapies. “Understanding how the molecular interactions that stabilize condensates can be fine-tuned is important when designing drugs to inhibit phase-separation or to dissolve condensates before they can get to the aberrant stage. If there is a reentrant phase, you need to ensure that the drug does not invoke changes that move the proteins back into a phase-separated regime,” explains Joseph. “We also found that some cancer-related proteins also display this transition behaviour, so our work could be relevant in developing cancer therapies,” adds Krainer.
Collaborative methods
The researchers used a combination of experimental and computational techniques to conduct their investigation. Krainer and Welsh are in the Knowles research group, and conducted their experiments in the Sir Rodney Sweetnam Laboratory
“We used advanced fluorescence imaging using a fluorescence microscope with a high sensitivity camera to study the proteins,” explains Welsh, who is a second-year postgraduate student. “Two of the proteins we studied, known as FUS and TDP-43, are thought to transition from liquid condensates to solid-like states, and this transition may be implicated in ALS. This shows how a single protein sequence can behave massively differently in terms of phase separation,” he says.
Joseph, working in the Collepardo research group based in Chemistry, Physics, and the Department of Genetics, carried out computer simulations to quantify the relative strength molecular interactions, using PMF (Potential of Main Force) calculations. All the simulations were conducted on the supercomputers of the Cambridge Service for Data-Driven Discovery.
Joseph says she is especially proud of the collaborative aspect of the research. “We’d have sit-down meetings to discuss their results and think about the best type of simulations to determine if their hypothesis was true or if we could come up with a better hypothesis.”
“I’ve always worked on purely simulation-based papers, and it was wonderful to get the opportunity to work alongside the experimentalists and get a feel for how they work. Having multidisciplinary teams is what is going to help us answer these questions – being able to see the problem from many different viewpoints, using different types of thinking and different techniques that can complement each other very well.”
Krainer agrees.“You can describe the phenomenon with experiments, but with computational tools you can really get answers on a molecular scale. I wouldn’t put one above the other – they are on one continuum. As a scientist with a background in molecular biosciences, I am proud that this study really embodies this multi-disciplinary character – it’s really fascinating to see how these areas complement each other.”
As a postgraduate student, Welsh is excited about the discoveries. “I think it’s a great opportunity to better understand the generality of the driving forces behind phase separation. On a broader scale we were also looking at the molecular grammar of liquid- liquid phase separation – trying to see what exactly in the proteins, in terms of their molecular makeup, causes them to behave the way they do in undergoing phase separation – we’re learning the language encoded in the specific amino acids, proteins, and domain structures – it’s like putting together letters, words and sentences.”
Origin of life
And what about the origin of life? “That’s another cool hypothesis stemming from our work,” says Joseph enthusiastically. “In the origin of life, the higher concentrations of salt present in the oceans at the time could have facilitated formation of protocells via protein phase separation to keep selected biomolecules phase-separated.” Krainer agrees: “The implications for evolution and the origin of life on earth in high salt bearing waters are fascinating.”
Research
Krainer, G., Welsh, T.J., Joseph, J.A. et al, Reentrant liquid condensate phase of proteins is stabilized by hydrophobic and non-ionic interactions, Nat Commun 12, 1085 (2021).