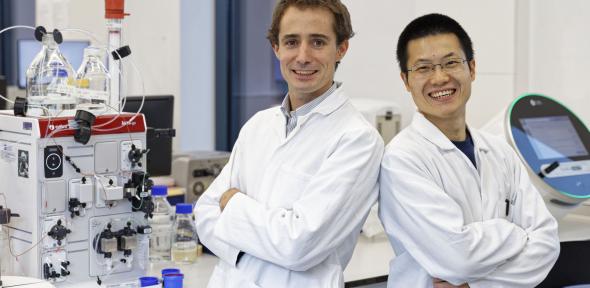
Dr Pietro Sormanni (left) and Dr Xing Xu in the lab taken by Nathan Pitt, ©University of Cambridge
Bespoke antibodies designed and tested in the lab are a promising tool to speed up drug design.
As research becomes more specialised and niche, we need groups to actively take disparate streams of knowledge and weave them together. Take antibodies and computer models. The Sormanni lab is developing a computational pipeline to simplify antibody discovery.
Antibodies are key tools to address questions and enable discoveries in biomedical research, are the workhorses of most diagnostic assays, and are increasingly used to treat a wide range of diseases as therapeutics, including cancer and neurodegeneration.
The current main method to discover new antibodies for a given target is to immunise animals, which has moral, time, and expense drawbacks. This new pipeline draws from a huge database of interacting fragments and it is much faster than conventional laboratory-based approaches.
Dr Mauricio Aguilar Rangel, first author of this research, said “We are excited about the results of our approach, as it opens the door for studying new targets of biological relevance. But most important, we see this as an important stepping-stone for developing a more comprehensive way of designing antibodies in silico”.
A perfect antibody
The computer initially scans the target that scientists want to address, for example, a cancer cell receptor or a viral protein. Then, the computer finds candidates that could bind to the chosen target and ranks them based on their compatibility with the target and on other properties. For example, their solubility. The scientists then take the top candidates and test their binding to the target in the lab.
A perfect antibody will attach itself only to its target and not to other proteins or surfaces. For example, in antibody-based anti-cancer therapy, the antibody latches onto the cancerous cell and either stifles its growth or destroys it whilst leaving healthy cells alone.
“We are aiming to make this pipeline time and cost-efficient so that it is simple to use in routine laboratory settings,” comments Dr Xing Xu, a research associate in the Sormanni lab, who is working on the next phases of this research.
When the computer software suggests an antibody, the antibody should be a potential lock to the target site. However, so far the locking is relatively weak. It is thus scientists like Xing’s job to refine it further so that the antibody strongly binds to the pre-determined surface. If this can be achieved, one can easily acquire an antibody with a high binding affinity to the pre-selected surface, which in most cases is a functional area related to the development of diseases.
From screen to bench
Starting from a computer-designed antibody, Xing deploys methods of in vitro directed evolution to optimise it further. This approach mimics evolution in a test tube, and starts with the construction of a library of variants of the original antibody, by introducing mutations on its binding surface. Then, subsequent rounds of screening are used to isolate from the library those variants that bind most tightly to the target. The result is a new antibody with improved binding characteristics over the one designed by the computer, but still binding to the same region selected with the software.
“Instead of the conventional antibody, we work with a specialised format, called a nanobody, which is around ten times smaller of the size of a full-length antibody. These small bullets have more versatile uses and are potentially useful for developing into a drug to target these hard-to-reach proteins,” Xing adds.
“When it comes to the blood-brain barrier, the antibodies inside our bodies cannot penetrate it effectively, which means that it is complicated to treat conditions in the brain. So, that is one reason why we are working with nanobodies.”
“Plus, nanobodies are naturally more stable and can be easily produced in single-celled organisms, facilitating the downstream development in a large-scale for research, diagnostics and therapeutics.”
This article first appeared in Chem@Cam Issue 65.