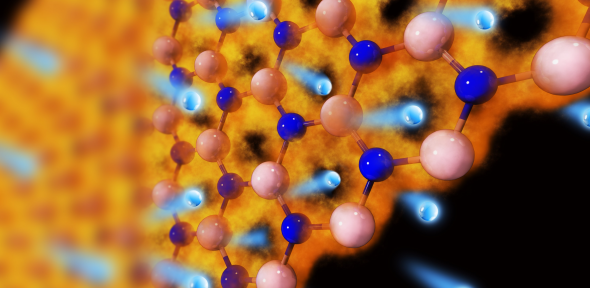
Unprecedented control over the inner workings of graphene.
The ICE Group at our department has played an important role in research led by the University of Manchester that will improve the ability to control the conductivity processes in graphene. This international collaboration was published today in Nature.
Graphene is a material made of a single layer of carbon atoms arranged in a hexagonal lattice. It is a tough, flexible material with high electronic conductivity which makes it useful in batteries and is of particular interest in renewable energy research such as rechargeable batteries, solar panels and semiconductors.
Now the researchers have shown that they can use electric fields to control protons as they pass through an atom-thick layer of graphene.
“This could enable new ways to improve processes at the base of many renewable technologies, such as hydrogen fuel cells,” said Flaviano Della Pia, a PhD student at our department who worked on the computational side of the research.
Controlling proton transport
An atom-thick layer of graphene is like a tiny chain-link fence: large particles, such as gases, are like basketballs that can’t travel through the fence but small particles, such as protons, are like marbles and fit through the gaps in graphene’s structure. This process is called proton transport and is essential in technologies such as batteries, renewable energy, and water-splitting devices.
The second process that involves protons as they move through a layer of graphene is hydrogenation, which is how they stick to the graphene. These two processes are normally linked together.
The researchers at the University of Manchester manipulated an electric field to decouple these two processes to control them separately. They used a device called double-gated graphene, that can independently control the electric field and the number of electrons in graphene, to disentangle these two processes and speed them up.
The ICE Group
The ICE Group confirmed the Manchester team’s findings by building a computer simulation to examine the system in microscopic detail.
“This research is scientifically fascinating and also of direct relevance to future energy technologies. Through simulation we are able to obtain atomic-scale insights and understanding into the phenomena measured,” said Angelos Michaelides, the department’s 1968 Professor of Chemistry.
Della Pia made a computer simplified model representing the experiment and examined the relationship between the electric field, the level of hydrogenation, and the energy barrier on the protons. The barrier the proton feels when it travels across the graphene layer is lowered when there is an electric field or if the levels of hydrogenation are increased.
“In our simulations, we show that the barrier that the proton feels when it goes across graphene is lowered both when we apply an electric field and when we increase the level of hydrogenation, as well as when these two processes happen at the same time.
The microscopic insight from atomistic simulation is in agreement with the trends observed in the experiment.”