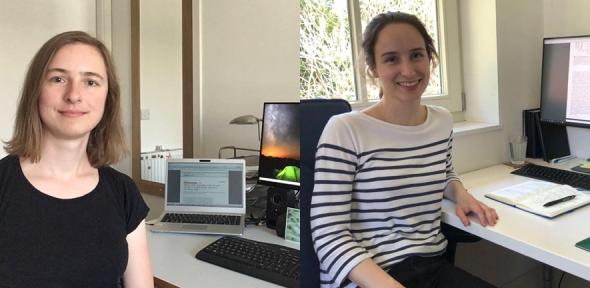
Co-authors Märker and Rinkel working from home during the Covid-19 lockdown
Researchers here have taken another small step forward towards commercially viable lithium metal batteries - and a large and exciting step forward in the understanding of these systems.
In research published in Nature Communications, Professor Clare Grey and colleagues report how they used a very old technique – but in a new way - to enhance NMR analysis of lithium-ion batteries, and particularly of the passivating layer that grows on the lithium metal in a battery, called the solid electrolyte interphase (SEI).
The development of longer lasting, higher energy density and cheaper rechargeable batteries is a major technological challenge. Lithium metal is considered the ultimate high-energy-density battery anode material, so one strategy to increase energy density is to use lithium metal in place of graphite in a lithium metal battery. “The reason people are interested in lithium metal is because it has a the highest energy density of all materials,” said Didi Rinkel, a PhD student in the Grey research group, and one of the paper’s co-authors.
However, a commercially viable rechargeable battery with a lithium metal anode has yet to be developed, partially because the use of lithium metal has been limited by dendrite formation and associated safety risks. Understanding how the SEI layer relates to the formation of lithium dendrites is seen as key to the development of lithium metal battery anodes.
“When a lithium-ion battery runs, the lithium ions move across the battery from the negative electrode to the positive electrode during discharge and back again during charge. You would like all the ions to deposit back on the Li metal anode in one flat layer, but they don’t – instead, they preferentially deposit at certain sites where they form structures called dendrites,” said postdoctoral researcher Dr Katharina Märker, also a co-author on the paper.
Taking up the explanation, Rinkel continued: “The dendrites are very thin, long lithium metal structures. If a dendrite grows from one side of the battery all the way to the other side, this can cause the battery to short circuit and even catch on fire.” The formation of dendrites is believed to be affected by the SEI, but this relationship is still poorly understood.
Professor Clare Grey has pioneered the use of NMR spectroscopy to study batteries in situ, and significant strides have been made in studying the SEI with NMR spectroscopy, in addition to other techniques such as X-ray photoelectron spectroscopy and time-of-flight secondary-ion mass spectrometry. However, because traditional NMR lacks sensitivity and selectivity, the nature and distribution of chemical components in the SEI and how they are related to dendrite formation remain unclear.
One way to solve these issues is by using dynamic nuclear polarisation (DNP) to boost signal intensities and enhance the NMR analysis. In typical exogenous DNP experiments a solution of organic radicals is added to the sample, and the higher polarisation of the free electrons in the radicals is transferred to the nuclei using microwave irradiation. DNP can therefore provide important structural information. But DNP also has its limitations: it generally requires cryogenic sample cooling, which is costly, and it is not selective for the interface between the metal and the SEI.
Professor Clare Grey
“In the 1950s, researchers first achieved DNP enhancements in NMR studies of Li metal and other compounds,” said Märker. “However, as NMR developed and began to use much higher magnetic fields, it was not possible to generate microwaves at high enough frequency and power required for DNP, and the technique they used fell out of fashion.”
However, with the development of commercially available gyrotron oscillators, it is now possible to generate the higher frequency microwaves which are required when working at the high magnetic fields employed in modern NMR. Building on the DNP experiments first performed on lithium metal more than 60 years ago, but using the much higher frequency microwaves now available, the team were able enhance the nuclear magnetisation via the same Overhauser effect originally used in the 50s.
In the Overhauser effect, the electrons in the lithium metal become the source of the polarisation, allowing selectivity for the metal/SEI interface. This eliminates the need for a solution of organic radicals, thus avoiding the risk of introducing impurities or altering the nature of the SEI. “The SEI is very fragile, and has the potential to react with the radicals that have to be added in typical DNP experiments, which could alter the nature of the SEI,” said Rinkel. “Also, in typical DNP experiments, you look at the SEI from the outer layer, but with this mechanism we could look at the SEI directly from the lithium metal,” said Rinkel.
“This new technique is important, because we’ve shown that we were able to selectively look at the SEI close to the lithium metal. Everyone tries to study the structure and the composition of the SEI layer, but most people do it from the outside in, mainly looking at the outer layer of the SEI. But since this mechanism uses the electrons in the lithium metal, this technique allowed us to look for the first time at the buried side of the SEI and view it much more clearly.”
The researchers also used magic angle spinning (MAS) to produce higher resolution signals. The NMR signals from solids are generally broadened by orientation-dependent interactions. Spinning the sample at the magic angle averages out the interactions, leading to sharper NMR signals.
Under MAS, the researchers were able to achieve significant enhancement of the Li NMR signal of cycled lithium metal anodes at room temperature. They then harnessed this to investigate the interface between lithium metal and the SEI. Using this process, the researchers were able to selectively enhance by about an order of magnitude individual NMR spectra of the SEI (lithium, hydrogen and fluorine) to reveal their chemical nature and spatial distribution.
Because the chemical composition and structure of the SEI is highly dependent on the electrolyte system used, they also made a comparison between samples with and without the common additive fluoroethylene carbonate (FEC), which has been shown to yield a more uniform lithium deposition and improved electrochemical performance.
The team also concluded that although resolution is reduced, appreciable enhancements can also be achieved by this process under static conditions, that is, when not using MAS.
‘This is good because it means we could do in situ experiments, where we can observe the battery while it is actually working,” said Märker. This could allow for further study of SEI formation and growth, one of the fundamental open questions regarding the SEI.
Such in situ studies would also benefit from the fact that the DNP experiments can be conducted at room temperature – which is compatible with battery operation and also adds a cost-saving factor. “Nowadays, most DNP-enhanced NMR experiments are done at very low temperatures of 100 K or below, only just a bit above the temperature of liquid nitrogen, which maximises the signal enhancements but is very costly,” explained Märker.
The researchers hope to apply this new technique to further experiments. “We would like to extend this to other battery chemistries that still use metals -- we expect that this mechanism can be used for most other metals – for example, we could use it to study Sodium (Na) batteries,” said Rinkel. “Sodium is much more abundant than lithium, and could make batteries cheaper. “
This process also has possible other applications in solid state chemistry and physics. “This new technique paves the way for more ambitious room temperature in situ studies of batteries, and the selective enhancement of metal-solid interfaces in a wider range of systems,” said Märker.
Professor Clare Grey said: “We are eagerly awaiting the delivery of our new DNP machine later on in the year, to use this novel approach to dig even deeper into the mechanisms thatcontrol Li dendrite formation - in and ex situ - and to probe the structure and dynamics in a range of metallic/diamagnetic interfaces in a much wider range of systems”
Selective NMR observation of the SEI–metal interface by dynamic nuclear polarisation from lithium metal, Nature Communications, Volume 11 2224(2020), Michael A. Hope, Bernardine L. D. Rinkel, Anna B. Gunnarsdóttir, Katharina Märker, Svetlana Menkin, Subhradip Paul, Ivan V. Sergeyev & Clare P. Grey.