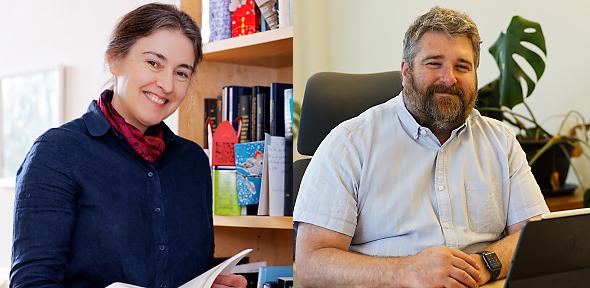
Professor Dame Clare Grey and Professor Oren Scherman, courtesy Chemistry Photography
In research published in Nature, scientists from the Scherman and Grey research groups demonstrate a path to batteries that do not contain rare metals and are stable in the air.
The researchers show how a ‘custom-built’ class of metal-free pyridinium molecules can act effectively as charge-carrying electrolytes in redox-flow batteries, even when exposed to air, which has potential for more stable and lower-cost batteries.
PhD student and co-first author Mark Carrington said: “The air stability would give us a new capability to create grid-scale energy storage which can be used under harsh conditions or in remote locations. It could be used to power cities and to help mitigate the intermittent nature of renewable energy.”
Carrington is jointly supervised by the Director of the Melville Laboratory and Professor of Supramolecular & Polymer Chemistry Oren A. Scherman and Royal Society Professor Dame Clare Grey.
Co-first-author Dr Kamil Sokolowski, a senior postdoc in the Scherman group, said: “We have developed a new family of one hundred percent metal-free molecules that can store, transfer, and exchange electrons in the presence of air. The technology is scalable as well as safe to transport, store and use.”
The team also describe how to predict the properties and performance of the molecules, so they can be ‘made-to-measure’ for a particular use.
Grid-scale energy
“One of the biggest challenges we face now is how to create the large-scale storage of energy from intermittent energy sources like wind and solar,” said Grey, who is a co-corresponding author. “We need batteries that are large enough and last long enough for the grid, say 20 to 40 years.”
Redox flow batteries (RFBs) are one of the most promising solutions for this challenge. RFBs are typically used for grid energy storage, for example attached to power plants or electrical grids, because they offer larger capacity in comparison to conventional solid-state batteries.
Like other types of batteries, RFBs use electrolytes to support charge transfer between the battery’s electrodes to store and supply electric energy. However, unlike conventional batteries, which store charge in solid electrodes, RFBs store charge in solution-phase redox active electrolytes. The ability to store energy in this form gives greater flexibility to the system design, which could ultimately lead to lower costs and improved safety.
However, one of the major disadvantages of existing RFBs is that they use metals such as vanadium as electrolytes. The researchers show that using the non-metal, pyridinium-containing (organic) molecules as electrolytes has the potential not only to improve the energy density of RFBs, but also to make them more sustainable.
Stable in air
Most RFBs are based on liquid electrolytes that are pumped through the system on separate sides of a membrane and degrade when exposed to air. One of the major advantages of the pyridinium-based molecules reported by the researchers is that they have potential to work in the air.
“We now have a fundamental, molecular-level understanding of how air stability can arise, and it gives us the ability to design molecules that can act as the next generation of electrolytes to solve the world’s energy storage problems,” said Scherman, a co-corresponding author.
Sokolowski added: “The molecules exist as a powder which is air stable and can be stored under ambient conditions, so preparation of the electrolyte solution is as simple as dissolving the molecules in a saline solution.”
Capacity fade
A major barrier to extending battery life that we are all familiar with is ‘capacity fade,’ which is when the amount of charge stored begins to decrease with repeated cycling.
This happens when the capacity of the charge-carrying molecules to hold electrons is reduced through degradation or decomposition. A major decomposition route is through exposure to oxygen in air, which causes formation of reactive oxygen species (ROS), detrimental for electrolyte molecules and other battery components.
The researchers used an array of characterisation techniques such as nuclear magnetic resonance (NMR), electron paramagnetic resonance (EPR) and mass spectrometry to study the pyridinium molecules in flow battery conditions over thousands of cycles.
“We found the molecules were able to carry a substantial amount of charge,” said Scherman. “Over hundreds of cycles they don’t fall apart, they don’t decompose, and what’s unique is that they can form looser, non-covalent dimers, so they can still transport the electrons.”
Grey said: “Normally the charge-carrying molecules react with trace oxygen and form ROS, which leads to degradation. However, these pyridinium molecules form non-covalent dimers which can encapsulate carried electrons. They do not interact with dissolved oxygen and they don’t degrade.”
Made-to-measure
The researchers utilised a class of molecules developed by the Scherman group over the past decade that are composed of two pyridinium moieties on each side of a central core group. The central core and the attached groups at either end of the molecule can be changed as needed, to create an extensive library of molecules to work with.
“We compared many different types of pyridinium molecules. What we found is that certain species behave differently based on their structure, and because we have access to this broad array of molecules, we were able to create a simple descriptor to predict which molecular properties would lead to the formation of radicals, and when the radicals would lead to degradation or non-covalent dimerisation that protects the electrolyte,” said Scherman.
Building a better battery
“These electrolytes enable a completely new way of building batteries,” said Scherman. “They could make RFBs easier to manufacture and transport.”
Carrington said: “Existing classes of grid battery have a few remaining drawbacks that have inspired intense research efforts, particularly over the last few years. We expect that our findings could lead to a whole new design of grid batteries that are truly fit for purpose and are ready to be deployed.
Sokolowski said: “This has opened myriad possibilities for applications in many different areas where electron transfer is critical, like energy storage. Usually, these require the presence of certain metals and are highly air-sensitive. In contrast, our technology is metal-free and air-tolerant. We are hoping that this will open new pathways for the development of the next generation of batteries.”
“Coming up with molecules that are less susceptible to degradation from air and developing a descriptor for when they will degrade will lead to better batteries,” added Grey.
Further research
The Grey group has recently been awarded a Faraday Institution Industry Sprint grant for £240 thousand to continue investigating high voltage redox flow batteries for demanding applications and to scale the concept from lab scale to kilowatt hour scale.
Continued development of the core electrolyte and electron mediator technology is ongoing in the Scherman lab from both fundamental and commercial perspectives.
Grey said: “This work takes us one step closer to designing commercial redox systems.”
Research
Associative pyridinium electrolytes for air-tolerant redox flow batteries, M. E. Carrington, K. Sokolowski, E. Jónsson, E. W. Zhao, A. M. Graf, I. Temprano, J. A. McCune, C. P. Grey and O. A. Scherman, Nature, (29 Nov 2023), 623, 949-955.