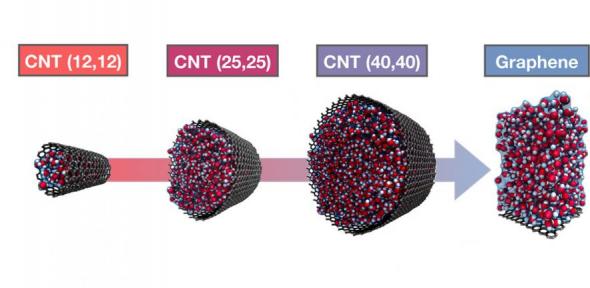
Interfaces define the common boundaries separating different types of matter. They are abundant in nature and find application across a range of disciplines, e.g., for biological function in the form of cell membranes. Modelling the properties of these interfaces – such as structure, dynamics, exchange, and reactivity, to name a few - constitutes an active area of research and is a central goal of the ICE group. Previous work has looked into characterising the controversial water/TiO2 system [1], understanding low-temperature water diffusion across metallic surfaces [2], and discerning the impact of interfaces on the dynamics of supercooled water [3]. Building on developments in materials modelling and improved computational resources, our current research employs state-of-the-art MD calculations and machine learning schemes to further our understanding. We are currently looking into the properties of catalytic surfaces and interfacial liquid-liquid reactivity.
An intriguing interface also arises in the form of water under nanoconfinement. Bulk water is notoriously complicated to study, but nanoconfined water presents a whole new challenge, manifesting a myriad of peculiar properties distinct from anything observed at the macroscopic level, e.g., unexpectedly low dielectric constants, enhanced rates of proton dissociation, and the possibility for near-frictionless flow along carbon nanotubes. The ICE group has led the way in investigating this unique behaviour, with recent studies looking to quantify the disparity in friction between carbon and boron nitride nanotubes [4] as well as constructing a first-principles phase diagram for monolayer water [5]. Citing applications in water desalination, blue energy harvesting, and biological cells, nanoconfined water presents both a stimulating and an important challenge for our future research. We are currently looking into the impact of explicit material choice on the properties of our confined systems as well as probing molecular reactivity under confinement.
References:
[1] H Hussain, G Tocci, T Woolcot, X Torrelles, CL Pang, DS Humphrey, CM Yim, DC Grinter, G Cabailh, O Bikondoa, R Lindsay, J Zegenhagen, A Michaelides, G Thornton – Nature materials (2016) 16, 461.
[2] W Fang, KM Meyer Auf der Heide, C Zaum, A Michaelides, K Morgenstern – Nano Letters (2021) 22, 340.
[3] P Gasparotto, M Fitzner, SJ Cox, GC Sosso, A Michaelides – Nanoscale (2022) 14, 4254.
[4] FL Thiemann, C Schran, P Rowe, EA Müller, A Michaelides – ACS nano (2022).
[5] Venkat Kapil, Christoph Schran, Andrea Zen, Ji Chen, Chris J. Pickard, Angelos Michaelides - arXiv:2110/14569 (2021).